
Electronics board. Image: anyaivanova/Shutterstock
Another week, another graphene breakthrough. Researchers in Austria have managed to demonstrate that the wonder material is excellent at carrying huge currents on the nano scale.
Of late, graphene has shown itself to be not only an excellent malleable sensor, but a possible solution to solving our overabundance of CO2 in the atmosphere.
A new breakthrough has shown graphene’s ability to transmit huge amounts of electric current at a microscopic level, which could add to its possible future in advanced electronics.
The international research team led by Prof Fritz Aumayr from the Institute of Applied Physics at TU Vienna was able to demonstrate that the electrons in graphene are extremely mobile and react very quickly.
This is achieved by impacting xenon ions with a particularly high electric charge on a graphene film that leads to a large number of electrons being torn away from the graphene in a very precise spot.
Yet within a matter of femtoseconds, the electrons had been replaced within the material, resulting in very irregular, extremely high currents.
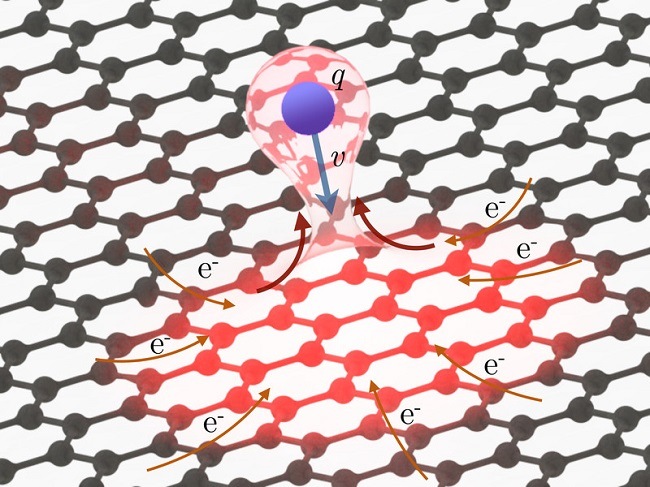
The reaction resulting from the surge in current in graphene. Image: TU Wien
Astounding results
“The xenon ion penetrates the graphene film, thereby knocking a carbon atom out of the graphene – but that has very little effect, as the gap that has opened up in the graphene is then refilled with another carbon atom,” said Elisabeth Gruber of the research group.
“For us, what is much more interesting is how the electrical field of the highly charged ion affects the electrons in the graphene film.”
Emphasising the weirdness of the sudden transformation of xenon ions into positive ones, Gruber’s colleague Richard Wilhelm described the process as “astounding”.
“What you would expect to happen now is for these positively charged carbon ions to repel one another, flying off in what is called a Coulomb explosion and leaving a large gap in the material.
“But astoundingly, that is not the case. The positive charge in the graphene is neutralised almost instantaneously.”
The end goal for the team’s research is to develop ultra-fast electronics using the single atom-thick material, particularly in connecting optical and electronic components.
The team’s research has been published in Nature Communications.