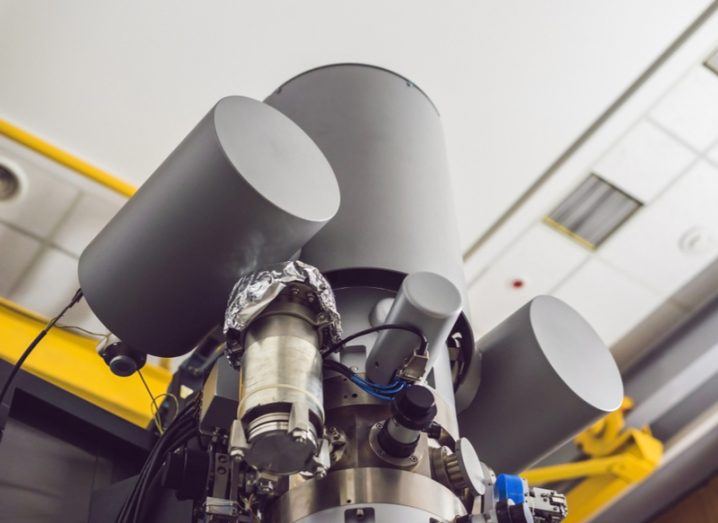
Image: Elizaveta Galitckaia/Shutterstock
In yet another graphene breakthrough, researchers have created an atomic-scale petri dish to help solve a glut of nanomaterial mysteries.
Whether it’s a humidity sensor or making ultra-efficient smartphones, the atom-thick material graphene promises an awful lot for something almost invisible to the naked eye.
However, harnessing these capabilities is a lot easier said than done, particularly when we’re trying to create something at the nanoscale.
The biggest hurdle is controlling growth and degradation at the atomic scale, but a new breakthrough from the University of Manchester might overcome this with the creation of a graphene petri dish allowing for nanomaterials to be observed in liquids.
Our existing means to analyse individual atoms requires scanning/transmission electron microscopy, which needs a high vacuum, but the structure of materials at room temperature in a vacuum can significantly differ from that in their normal liquid environment.
In a paper published to Nano Letters, the team revealed that graphene and boron nitride can be combined to create a perfect nano petri dish.
Any liquid samples inside this minute dish can be imaged with single-atom sensitivity and it is also possible to measure their elemental composition at the nanometre-length scale.
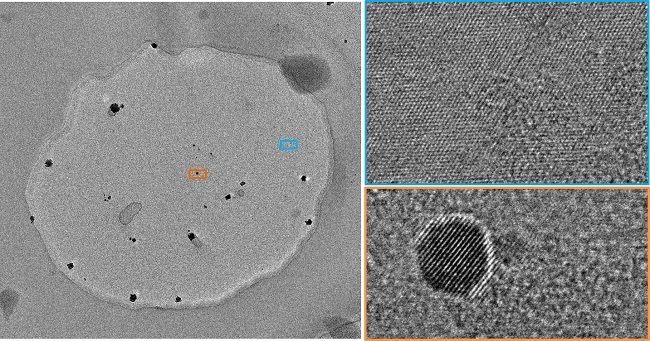
The graphene petri dish containing individual atoms. Image: University of Manchester
The dish is put together with building blocks called graphene liquid cells. Because the so-called wonder material is strong enough to protect a sample in a high vacuum yet also transparent, it does not compromise the electron beam that analyses the samples.
“Unlike some previous designs, our graphene liquid cells allow us to image the atoms for many minutes,” said the study’s lead author, Daniel Kelly. “We were even able to resolve individual atoms in water and observe them dancing under the electron beam.”
Using these new graphene liquid cells, the team was also able to significantly improve the quality of elemental analysis in liquid cells.
This new ability to monitor tiny concentrations over such small length scales is a necessity for the increasingly complex chemical structures of high-performing nanocatalysts, the team said.